Olivocochlear Reflex Strength and Resistance to Acoustic Injury Stephane F. Maison and M. Charles Liberman Department of Otology and LaryngologyHarvard Medical School and Eaton-Peabody Laboratory Massachusetts Eye and Ear Infirmary Boston, MA
1. Introduction
Overexposure to intense sound can cause permanent damage to the ear and noise-induced hearing loss (NIHL). NIHL studies, in humans and animals, have repeatedly documented a high degree of inter-subject variability: i.e. some individuals have “tough” ears and others have “tender” ears, even when sound exposure is very carefully controlled.1 The example on the next slide shows the NIHL range for 12 identically exposed guinea pigs. Despite considerable research, a safe and effective predictor of acoustic vulnerability has never been described. Notably, the vulnerability to minimally traumatic exposures, which produce only temporary hearing loss, does not predict vulnerability to permanent NIHL.2
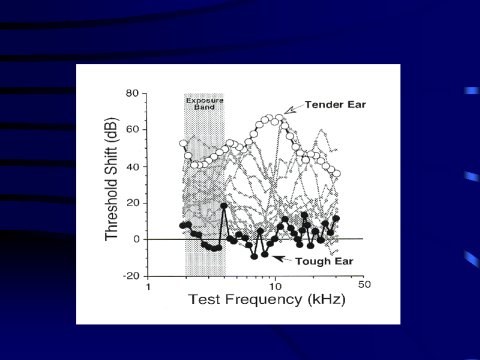
As shown above, unilateral de-efferentation increases permanent NIHL on the cut side,3 suggesting that the olivocochlear (OC) system may protect the ear from acoustic injury. Studies in both human and animals show that the strength of the sound-evoked OC reflex varies among individuals.4 These observations suggest the hypothesis that inter-subject differences in OC reflex strength underlie the differences in vulnerability to acoustic injury. If true, an OC reflex test could be used to screen for individuals with tough vs. tender ears.
2. Methods
- Animals: Albino guinea pigs weighing 400-550g were screened for normal cochlear function by measuring DPOAEs at 2f1-f2 for f2=10kHz. Animals passing the screen were arbitrarily assigned to one of three sound exposure groups (see below), or to the control group (n=14 ears) with no acoustic overexposure. OC reflex strength was measured twice (see right column) before exposure.
- Acoustic Overexposure: After reflex testing, animals were exposed free field, awake and unrestrained, in a small reverberant chamber. Acoustic trauma consisted of a 4-h exposure to an octave band noise presented at 109dBSPL. Exposure levels, measured at four positions within each cage, varied by less than 0.5dB. Animals were allowed to recover for 1 week post exposure to allow for recovery of temporary threshold shifts.
- PTS Measurements: For the final test of cochlear thresholds, animals were anesthetized with sodium pentobarbital (i.p.) and fentany/droperidol (i.m.). The cartilaginous ear canals were severed, bullas opened, and CAP was recorded via a silver wire on the round window referred to the tongue. CAP threshold was measured under computer control in response to 5-ms tone pips (10/s) with 0.5ms rise fall. At each SPL, 32 responses were averaged. Threshold was defined as the sound pressure required to produce a peak-to-peak potential of 6V.
- OC Reflex Strength was assayed in awake guinea pigs on two test days, separated by 1 week. The assay measured, with fine time resolution, post-onset adaptation of the DPOAE at 2f1-f2. OC activity evoked by the primary tone feeds back to cause this adaptation.5 At each test session, data were obtained at 176 level combinations (16 f2 levels X 11 f1 levels): f1 level (P1) was fixed at one of 11 levels (75-85 dB SPL), at each P1 level, f2 was varied in 16 steps from P1-15 to P1. One such run (P1=80dB, 65< P2 <80) is shown on the next slide for two different animals. The frequency of f2 was always 10kHz (f2/f1 = 1.2). Post-onset adaptation is sometimes positive, and sometimes negative: to compute a single value of OC reflex strength from all 176 measures, the difference between the maximum and minimum adaptation for all 16 f2 levels at one f1 level was determined and these values were averaged over all 11 f1 levels.
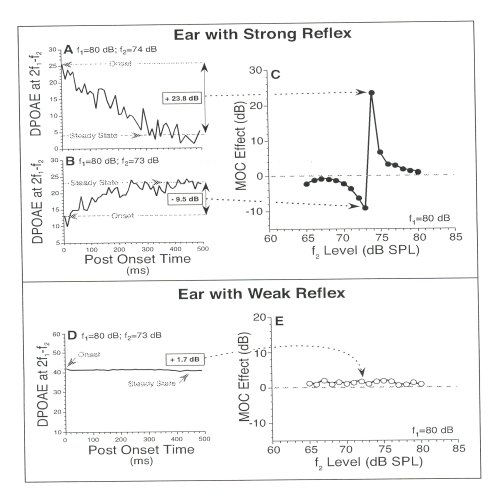
3. Results
- A DPOAE-based assay gives reproducible measures of OC reflex strength in awake animals.We applied our non-invasive measure of OC reflex strength to 36 awake guinea pigs in each of two test sessions, several days apart. The results of the 1st test session were highly correlated with the 2nd session: i.e. Some animals had reproducibly strong reflexes, while others were reproducibly weak. As shown on the following image, the range of test results can be used to arbitrarily divide these 36 experimental animals into those with weak, intermediate, or strong OC reflexes.
- OC reflex strength predicts PTS magnitude.When grouped according to pre-exposure OC reflex strength (next slide), animals with weak reflexes had the largest permanent threshold shift (PTS), while animals with strong reflexes had the smallest. Intergroup PTS differences between the strong and weak were statistically significant (p<.03 by two-way ANOVA) for all 3 noise-exposure groups. Animals with intermediate reflex strength showed intermediate values of PTS, except for Group C, where they were not significantly different from weak reflexes. Error bars indicate standard errors of the mean. CAP data were obtained from both ears of each experimental animal.
- Correlation between PTS and OC reflex strength was best at test frequencies near the PTS peak.The correlation between PTS and OC reflex strength was analyzed for each exposure group at a number of PTS test frequencies. One example (PTS at 4.02kHz vs OC reflex strength for the 24 ears in Group A) is shown at the left (r = 0.78, p<.001). At the right, correlation coefficients are shown for all test frequencies at which the mean PTS was >20dB: Note that the correlations are high near the test frequencies at which PTS was maximal, as indicated by arrows.
4. Discussion
OC effect of the type measured in our DPOAE assay are mediated by the medial (M)OC component projecting to OHCs, rather than lateral (L)OC component which projects primarily to dendrites of auditory nerve fibers. The most compelling evidence for this is that, in a mouse lacking the 9 cholinergic receptor expressed in OHCs but not in afferent neurons, all OC-induced suppressive effects disappear, including those on DPOAEs.6
Nevertheless, the strong correlation observed here between MOC reflex strength and vulnerability to acoustic injury does not prove causation. Indirect evidence suggest that high MOC feedback activity is correlated with high LOC feedback activity, although the peripheral effects of activating the LOC system are not yet clear.7,8 Thus, it is possible that high MOC reflex strength is indicative of a generally hyper-reflexive state, and that the cochlear protection is actually afforded by the LOC, rather than the MOC system.
Regardless of the mechanisms underlying OC-mediated protection, the correlation between MOC reflex strength and acoustic vulnerability provides a powerful non-invasive screen for individuals with “tough” vs. “tender” ears. MOC reflex strength can be measured in human subjects, based on OAE suppression by contralateral sounds.4 Furthermore, MOC reflex strength varies among human subjects. Thus an OAE-based test should also work in human populations to identify those most at risk for noise-induced hearing impairment, thus providing a strategy for reducing future injury.
5. References
1. Cody, AR & Robertson, D. Variability of nise-induced damage in the guinea pig cochlea: electrophysiological and morphological correlates after strictly controlled exposures. Hear. Res., 9, 55-70 (1983).
2. Ward, WD. The concept of susceptibility to hearing loss. J. Occup. Med., 7, 595-607 (1965).
3. Kujawa, SG & Liberman, MC. Conditioning-related protection from acoustic injury: effects of chronic deefferentation and sham surgery. J. Neurophsio., 78, 3095-3106 (1997).
4. Moulin, A., Collet, L. & Duclaux, R. Contralateral auditory stimulation alters acoustic distortion products in humans. Hear. Res., 65, 193-210 (1993).
5. Liberman, MC. et al. The ipsilaterally evoked olivocochlear reflex causes rapid adaptationof the 2f1- f2 DPOAE. J. Acoust. Soc. Amer. 1996: 99:3572-3584.
6. Vetter, DE. Et al. Role of 9 nicotine ACh receptor subunits in the development and functionof cochlear efferent innervation. Neuron 23, 93-103 (1999).
7. Liberman, MC. Physiology of cochlear afferent and efferent neurons: direct comparisons in the same animal. Hear. Res. 34, 179-191 (1988).
8. Liberman, MC. Effects of chronic cochlear de-efferentation on auditory-nerve response. Hear. Res. 49, 209-223 (1990). |